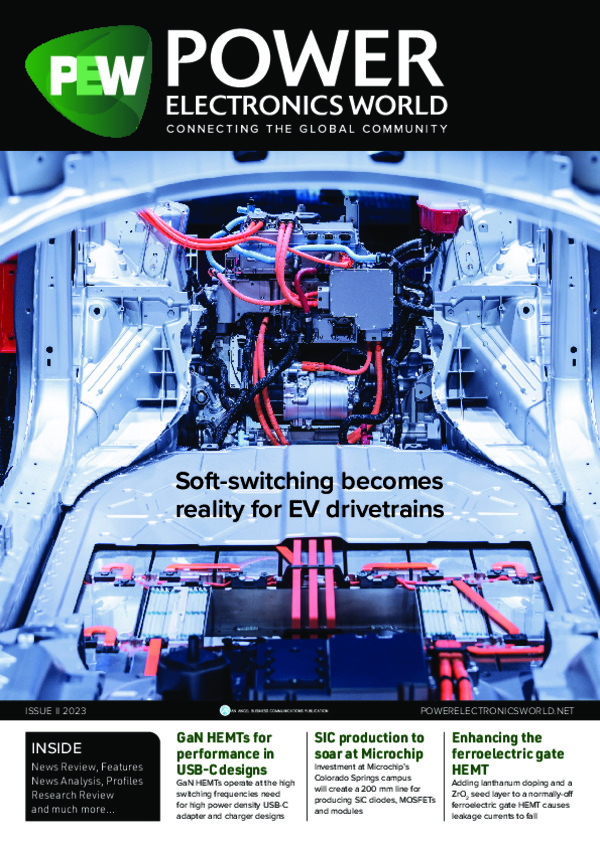
CS International: Championing a true heavyweight: Unleashing the promise of Ga₂O₃
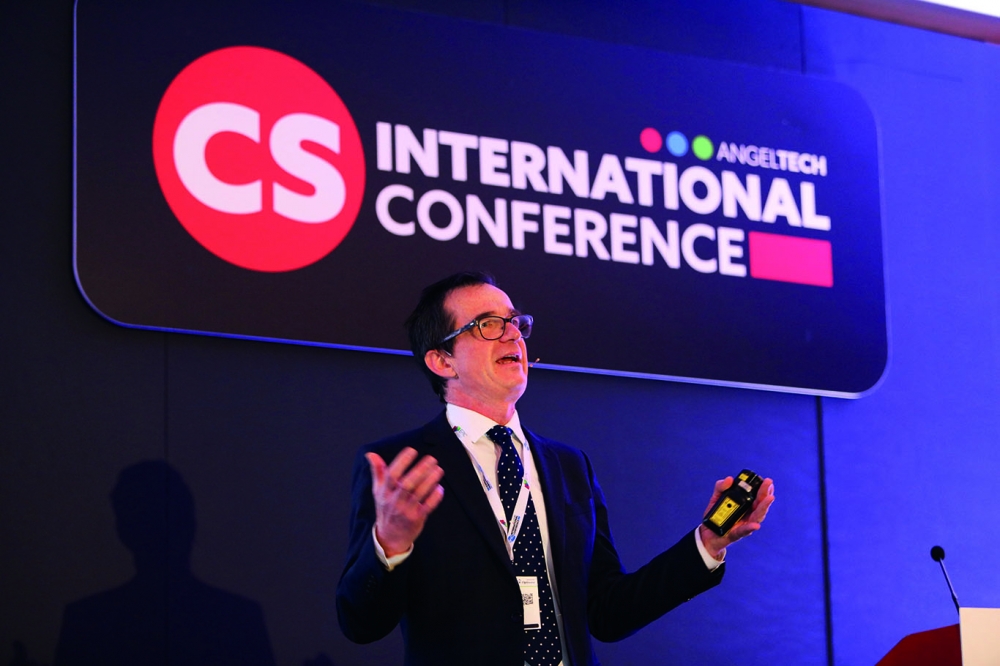
Blessed with an incredibly wide bandgap, shallow donors and the
capability of bulk growth from the melt, Ga₂O₃ is making a compelling
case as the most promising material ever for power devices.
BY RICHARD STEVENSON, EDITOR, CS MAGAZINE
Aside from a record number of delegates and a packed exhibition hall, those attending this year’s CS International will remember this meeting for the phenomenal level of interest in wide bandgap power electronics. Excitement in this sector is incredibly high, because sales of SiC and GaN devices and modules are set to rocket throughout the remainder of this decade, climbing from an annual revenue that now totals below $2 billion to around $18 billion by 2030. That’s great news not only for chipmakers, but also those making related materials, metrology tools and device design software.
A healthy uptake of these two new classes of power electronics with benefit humanity. There will be improvements in the efficiency with which electricity is converted between its AC and DC forms, and is stepped up and down. This leads to a reduction in the carbon footprint of numerous electrical systems. What’s more, electric vehicles will travel further on a single charge, helping to sooth concerns related to range anxiety, as well as making a more compelling case for switching away from the combustion engine.
But does the revolution in power electronics end with SiC and GaN? Or is there more to come?
It’s the latter, argued three presenters at CS International: Martin Kuball (pictured above), leader of the Centre for Device Thermography and Reliability at Bristol University; Akito Kuramata, CEO of gallium oxide substrate maker Novel Crystal Technology (NCT); and Heather Splawn, CEO of HVPE specialist Kyma Technologies. All made the case that compared with the middleweight duo of SiC and GaN, gallium oxide is a heavyweight with the capability to handle incredibly high voltages and provide switching at even higher efficiencies. These attributes make this oxide a very promising candidate for the ultra-high-voltage market, where it could be deployed for supporting the grid, handling the power produced by wind turbines and finding application in electric trains.
Akito Kuramata is CEO of Novel Crystal Technology, a provider of Ga2O3
substrates and epiwafers. The company plans to expand into the
production of diodes and transistors based on this oxide.
In terms of its physical prowess, Ga2O3 is best known for its very wide bandgap of 4.9 eV and a breakdown field that can reach as high as 8 MV cm-1. But how does it perform when benchmarked against the common yardstick for gauging the potential of power devices, Baliga’s figure of merit? Well, that depends on what’s considered. Initially, while it would appear that oxide is ahead of SiC and GaN by a considerable margin, it lags behind two other heavyweights, diamond and AlN. However, when one considers the depth of the dopants, a crucial factor in determining the capability of power devices, the β form of Ga2O3 comes out on top, thanks to relatively shallow dopants (see Figure 1).
Figure 1. Once the level of activity of dopants is considered, Ga2O3
emerges as the most promising of the ultra-wide bandgap semiconductors,
based on Baliga’s figure of merit. This graph is taken from Y. Zhang et
al. Semicond. Sci. Technol. 35 125018 (2020).
Another major asset of Ga2O3 is the relative ease of crystal growth. Like silicon, GaAs and InP, it can be grown from the melt, ensuring relatively easy production of substrates with low dislocation densities. That’s not the case for SiC, which tends to be produced by vapour phase transport; or GaN, which is yet to have a bulk growth technique suitable for producing native power electronic devices in volume.
Obstacles to overcome
Thanks to all these merits, devices made from Ga2O3 are already delivering impressive results. “The performance is already exceeding silicon carbide,” remarked Kuball during his presentation to delegates at CS International 2023. However, that does not imply that commercial success is assured, partly due to weaknesses associated with these devices.
Kuball did not brush aside these concerns, but discussed them head on. One weakness is a high density of defects in the material, with deep-level transient spectroscopy uncovering many different trap states in β-Ga2O3. More work is needed, as it is still largely unknown what the killer defects in this oxide might be.
Another concern is the low thermal conductivity of Ga2O3, which has led many to claim that this oxide will never be a viable material for power devices, due to overheating of the chip. But this issue can be addressed with engineering, with Kuball suggesting that the introduction of diamond beside the active region could suck heat away. It’s an approach his team have already used to improve the thermal management of GaN devices.
Figure 2. NCT intends to increase the diameter of the substrates it
produces, as well as branching out into the production of Ga2O3 diodes
and transistors. semiconductors, based on Baliga’s figure of merit. This
graph is taken from Y. Zhang et al. Semicond. Sci. Technol. 35 125018
(2020).
The addition of diamond is actually capable of offering much more than simply superior heat extraction. Work by Kuball’s team has shown that superjunction Schottky barrier diodes formed by filling trenches in n-type Ga2O3 with p-type diamond enables electrical control of the device. This is encouraging, because the lack of p-type doping of Ga2O3 is a major concern – and integrating other p-type semiconductors is a promising solution.
When any device is in its infancy, there will always be concerns over its reliability. Kuball and his co-workers have been investigating Ga2O3 trench FETs provided by the University of Cornell, with experiments finding failure at the dielectric, Al2O3. Efforts will now need to be directed at improving the interface between Ga2O3 and Al2O3.
Trench Schottky barrier diodes have also been produced by the Bristol team. Benchmarking of these devices, which do not have field plates, indicates a performance comparable to that of the other leading groups in this field.
In May 2022 Kuball’s team commissioned the first commercial Ga2O3 MOCVD reactor in Europe, an Agnitron Agilis tool. Since then they have been enjoying the opportunity to grow their own material, producing both Ga2O3 and Alx(Ga2O3)1-x epilayers for a variety of structures, including vertical devices.
Kuball explained that to improve the thermal performance of devices, they have investigated two-step growth on diamond. Transmission electron microscopy and scanning electron microscopy images of a 245 nm-thick layer of β-Ga2O3 have revealed a completely coalesced surface, and different competing crystalline orientations. The physical characteristics of this film are not that different from β-Ga2O3 grown on sapphire, leading Kuball to describe results so far as very promising.
Figure 3. For high-speed growth of Ga2O3 material with a large diameter,
edge-defined, film-fed growth delivers unparalleled results.
Options for boule growth
More insight into the growth of Ga2O3 boules came in the presentation from NCT’s Kuramata, who discussed both of the growth technologies that his company has employed to produce commercial material: edge-defined, film-fed growth; and vertical Bridgeman growth.
While this Japanese firm, based in Sayama, is better known for its production of substrates and epiwafers, it also has plans to produce chips and packaged devices. Kuramata presented a roadmap for the company, which included the introduction of 150 mm and 200 mm wafers within the next five years, and the launch of diodes and transistors in 2023 and 2025, respectively (see Figure 2 for details). The company’s diodes are intended to offer a cost performance exceeding that of the SiC Schottky barrier diode, while judged by the same yardstick, transistors are expected to have the upper hand over the silicon IGBT.
For the production of larger material, edge-defined, film-fed growth leads the way, with NCT already having reported the development of material with dimensions of 6 inches. “It’s the only way to produce large n-type substrates at present,” remarked Kuramata.
Figure 4. NCT uses the vertical Bridgeman process to produce Ga2O3 crystals with a very high quality.
Another merit of this film-fed growth approach is that it’s the fastest of all the Ga2O3 growth technologies – with a growth rate of 15 mm/hour, it’s three times as fast as a float-zone process, and considerable faster than growth by the Czochralski and vertical Bridgeman techniques, which are capable of only 2 mm/hour and 1 mm/hour, respectively.
With edge-defined, film-fed growth, engineers draw molten gallium oxide through a slit by capillary action, leading to growth on seed material (see Figure 3). This yields crystalline material with a plate-like geometry that has a defect density of typically around 103 cm-2.
As the Ga2O3 industry is still in its infancy, it’s not surprising that today there are no orders for 6-inch material. However, Kuramata is confident that once such orders arrive, NCT will quickly establish production of material of this size.
The Japanese outfit is also developing Ga2O3 boules produced by the vertical Bridgeman process, as this yields material with a very high quality. Growth involves a crucible made from a platinum-rhodium alloy and a (010) Ga2O3 seed crystal. By carefully controlling the motion of the crucible through the thermal gradient produced in the furnace, molten Ga2O3 is cooled to yield a crystalline boule. With this approach, NCT has produced 2-inch (010) substrates – claimed to be the largest size for (010) Ga2O3 substrates ever reported.
For the growth of epilayers, NCT employs HVPE, a technology transferred from Tokyo University of Agriculture. Working in partnership with Saga University, NCT has produced Schottky barrier diodes on a 100 mm β-Ga2O3 epiwafer, with chip sizes of up to 10 mm by 10 mm. For a 10 µm-thick layer, film thickness uniformity is ± 5 percent; and donors, at a concentration of 1 x 1016 cm-3, have a variation of ± 7 percent.
Diodes from this wafer with sides of 10 mm by 10 mm have a yield as high as 51 percent. Based on this figure, the killer defect density for the epiwafers is about 0.7 cm-2.
Using the vertical Bridgeman technique, NCT has produced 2-inch (010) substrates.
Championing HVPE
Another advocate of HVPE for gallium oxide high-voltage power electronics is the leader of Kyma Technology, Heather Splawn. She argued at this year’s CS International that this form of epitaxy produces material with a low cost and high performance, using high growth rates that are ideal for device manufacture. Splawn also pointed out that HVPE is inherently cleaner than MOCVD, due to chemically pure precursors and the absence of metal-organics, which contain carbon. Thanks to these assets, HVPE is capable of high-purity growth.
Kyma has launched to market a tool called Katharo, designed for the growth of Ga2O3 devices for high-power switching. This reactor is capable of accommodating wafers up to 200 mm in diameter.
While growth on such large diameters is still some way off, the company has realised encouraging results on smaller substrates. In HVPE reactors with 100 mm capability, excellent doping control is realised at epilayer thicknesses that can be more than 20 µm, according to Splawn. Her team has also deposited Ga2O3 epilayers with uniform doping and thickness on 50 mm wafers, with X-ray diffraction measurements indicating a very high level of crystal quality – linewidths are just 25-30 arcsec.
Using HVPE, Kyma has grown a 16.7 µm-thick layer of Ga2O3 on a 2-inch
native substrate that has a thickness variation of ± 3 percent. Values
for the difference between the concentration of donors and acceptors within this epilayer range from 2.7 x 1016 cm-3 to 5.8 x 1016 cm-3.
Material produced by Kyma has been used to produce some very encouraging device results. Breakdown fields in these devices are as high as around 5.5 MV cm-1, and Baliga’s figure-of-merit is in excess of 1 GW cm-2, a value very close to the theoretical limit for SiC.
Such results highlight the great promise of Ga2O3. With SiC and GaN seemingly assured of a bright future, it will be some time before this oxide makes a really big impact – both at CS International and within the power industry – but there’s no doubting that the omens are good.
Kyma’s CEO, Heather Splawn, advocates HVPE for HVPE – that’s hydride vapour phase epitaxy for high-voltage power electronics
Main image: Martin Kuball, chair of the Royal Academy of Engineering in Emerging Technologies and a physics professor at the University of Bristol, UK, is expanding his research into gallium oxide, thanks to the introduction of an MOCVD growth tool in his labs.