The Rise of GaN-Based Power Systems
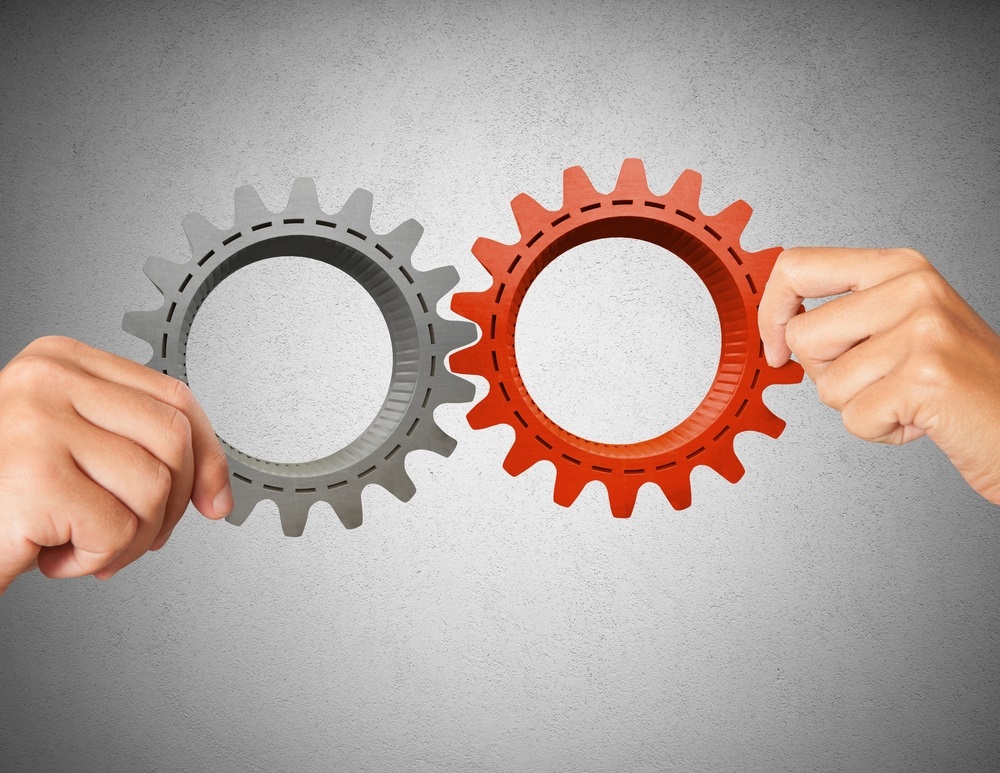
Higher-efficiency power conversion is critically essential to meet the needs of systems in areas of increasing reliance such as data centers, electric vehicles, renewable energy, industrial motors, and consumer electronics that are consuming ever more power. For example, industrial motors use 30 percent of the world's electrical energy. Data centers are forecasted to consume 5-10 percent our energy resources -- and with the growing number of IoT applications and devices and the arrival of 5G, the resources required to power them are adding more demand every day.
The significant benefits that Gallium Nitride (GaN) technology was expected to bring to the power electronics market are now being realized, especially in the industries mentioned above.
This article, Part 1 of a multi-part series, describes the growth of the GaN power market and the main application areas being affected. It gives a brief overview of the fundamentals of GaN technology and describes the similarities and advantages of GaN E-HEMTs compared to traditional silicon MOSFETs. It also provides examples of advanced E-HEMTs that are facilitating the rapid adoption of GaN designs in applications that require the highest possible efficiency.
GaN Market Overview
A report from Yole Développement projects the power GaN market to grow at a 79 percent CAGR over the next five years, and by 2020 to reach $460 million USD in revenue. According to the analyst firm, the most significant volume will come from the AC/DC and DC/DC power supply market (a predicted $250 million USD by 2020), with a significant portion addressing data center power.
Other key areas for GaN adoption are electric and hybrid electric vehicles and wireless power. Additional markets, including solar (PV), industrial motor drives, Class D Audio, UPS systems and consumer electronics, are also driving forces behind this growth (Figure 1).
Figure 1. Yole projection of GaN adoption growth by various markets by year.
As these market areas would suggest, GaN devices offer significant efficiency gains for power electronics designs that surpass traditional approaches.
Fundamentals of E-Mode GaN HEMTs
In their native form, GaN HEMTs power transistors are depletion mode (normally ON) devices. In power switching applications, d-mode devices typically utilize a cascode configuration to effect normally OFF operation. The complexity of cascode implementation of GaN transistors, essentially multi-chip modules, has limited the market acceptance of this type of product.
E-mode (enhancement-mode) HEMTs on the other hand, are conceptually similar to normally OFF silicon MOSFETs. They consist of three terminal devices with gate, drain, and source nodes. Similar to silicon MOSFETs, a positive voltage between gate and source on the HEMTs enables a high-electron-mobility path between the drain and source terminals (Figure 2). When the gate is held at or below source potential, the high-electron-mobility path is interrupted and no current flows between drain and source. Additionally, a GaN device's Qg, or gate capacitance, is lower by an order of magnitude or more than a silicon MOSFET device, which makes driving an E-HEMT device much easier.
Figure 2. Topology for e-mode GaN devices is conceptually similar to silicon MOSFETs.
The same circuit techniques used to drive traditional silicon MOSFETS can also be used with e-mode GaN HEMTs. In other words, the GaN device works much like a silicon MOSFET, only with much better switching and circuit performance. Additionally, GaN E-HEMT devices are closer to the "ideal switch" in power electronics applications because they do not have an intrinsic parasitic body diode that silicon MOSFETS do. Thus, there is no need for anti-parallel diodes.
Advances in E-Mode GaN
The desirability for the benefits of e-mode GaN has yielded advances in e-mode GaN HEMT technology such as the Gan Systems E-Mode product line. The benefits of these advanced devices include a more robust gate structure than similar devices, no DC holding current, and no complex gate diode PN junction. They switch on average four times faster and turn off at least two times faster than comparable devices on the market. By spending less time in the linear region, efficiency is greatly improved. Also, their lower gate capacitance means less energy is consumed driving the gate. The resulting energy savings applies right to the bottom line of increased power converter efficiency.
Not only can E-HEMT GaN devices upgrade many existing topologies, they enable new applications that were not possible with traditional options. For example, E-HEMTs can replace IGBTs in half-bridge hard-switching power converter circuits with drastic improvements in circuit performance. Moreover, E-HEMT GaN devices enable innovative topologies not possible with MOSFET devices such as the bridgeless totem pole topology (Figure 3). Silicon MOSFETs could never be used for these applications due to the limitations of QRR.
Figure 3. The Totem-pole topology possible with E-HEMT GaN delivers dramatic improvements in efficiency and size.
Benefits for Power System Design
As has often been observed, semiconductor advances are either the result of improvements in process or packaging. In the case of the GaN Systems E-Mode devices, both areas are evident. The work being done to increase the benefits of E-HEMT GaN devices include embedded die and advanced packaging and co-packaging of drivers and the GaN switches to minimize parasitic interconnections "“ offering functional modules and more.
The resulting benefits of using this are many:
· The need for heat sinking is reduced -- with opportunity to use the PCB as the heat sink "“ thus decreasing weight, volume, cost, size and assembly cost, and complexity of the device
· Increasing output power and/or switching frequency results in reducing the size of the magnetics, making them smaller and lighter
· Faster switching speeds and slew rates means the switching devices themselves spend less time in the linear region thus lower switching
· Also, higher operating temperatures are possible due to the wide bandgap construction and larger potential barrier structures
· Low parasitics, overall, and much lower capacitances, including gate capacitance, means that it is much easier to drive and lower gate drive losses
· Higher breakdown voltages (due to the overall wide bandgap construction) and higher current (due to higher carrier density and increased electron mobility) are possible
· Eliminating the parasitic diode means better circuit operation and fewer external components needed in addition to zero recovery charge in circuit operation
These device attributes translate to the benefits of reduced size and weight "“ to a quarter that of a silicon design, lower system cost and increased efficiency "“ all which results in lower total cost of ownership for the end user.
Industry Roadmap and Market Trends
Reducing size and weight, while increasing performance (including power density), is now a requirement for power system applications. In the transportation sector, for instance, some automakers have announced that they will discontinue internal combustion engines within the next several years. This means that the infrastructure for electric transportation systems will need to be rapidly built out. Moreover, electric vehicle rapid-recharging stations will need extremely efficient power electronics as well as on-board power electronic systems in the vehicles themselves.
Also, one of the biggest needs for efficiency will be in server farms and data centers, which are being built out at a tremendous rate to serve the insatiable need for communications and storage for big data, IoT, and general Internet traffic. In addition to saving energy, any increases in power density and efficiency are especially coveted since they translate directly into lower utility costs.
It's clear that the needs of the power electronics market aligns with the demonstrated benefits of GaN devices when incorporated into power electronics systems.
In part 2, we will further explore the technical details of applying e-mode GaN devices to effect innovative and highly efficient power conversion topologies.